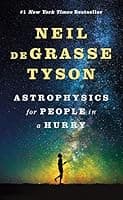
In the beginning, nearly fourteen billion years ago, all the space and all the matter and all the energy of the known universe was contained in a volume less than one-trillionth the size of the period that ends this sentence.
Conditions were so hot, the basic forces of nature that collectively describe the universe were unified. Though still unknown how it came into existence, this sub-pinpoint-size cosmos could only expand. Rapidly. In what today we call the big bang.
the four distinct forces we have come to know and love: with the weak force controlling radioactive decay, the strong force binding the atomic nucleus, the electromagnetic force binding molecules, and gravity binding bulk matter.
For the first billion years, the universe continued to expand and cool as matter gravitated into the massive concentrations we call galaxies.
Nearly a hundred billion of them formed, each containing hundreds of billions of stars that undergo thermonuclear fusion in their cores. Those stars with more than about ten times the mass of the Sun achieve sufficient pressure and temperature in their cores to manufacture dozens of elements heavier than hydrogen, including those that compose planets and whatever life may thrive upon them. These elements would be stunningly useless were they to remain where they formed. But high-mass stars fortuitously explode, scattering their chemically enriched guts throughout the galaxy. After nine billion years of such enrichment, in an undistinguished part of the universe (the outskirts of the Virgo Supercluster) in an undistinguished galaxy (the Milky Way) in an undistinguished region (the Orion Arm), an undistinguished star (the Sun) was born.
As less and less accretable matter remained in the solar system, planet surfaces began to cool. The one we call Earth formed in a kind of Goldilocks zone around the Sun, where oceans remain largely in liquid form. Had Earth been much closer to the Sun, the oceans would have evaporated. Had Earth been much farther away, the oceans would have frozen. In either case, life as we know it would not have evolved.
A mere sixty-five million years ago (less than two percent of Earth’s past), a ten-trillion-ton asteroid hit what is now the Yucatan Peninsula and obliterated more than seventy percent of Earth’s flora and fauna—including all the famous outsized dinosaurs.
This ecological catastrophe enabled our mammal ancestors to fill freshly vacant niches, rather than continue to serve as hors d’oeuvres for T. rex. One big-brained branch of these mammals, that which we call primates, evolved a genus and species (Homo sapiens) with sufficient intelligence to invent methods and tools of science—and to deduce the origin and evolution of the universe.
But what if the universe was always there, in a state or condition we have yet to identify—a multiverse, for instance, that continually births universes? Or what if the universe just popped into existence from nothing? Or what if everything we know and love were just a computer simulation rendered for entertainment by a superintelligent alien species?
People who believe they are ignorant of nothing have neither looked for, nor stumbled upon, the boundary between what is known and unknown in the universe.
the universe had a beginning. The universe continues to evolve. And yes, every one of our body’s atoms is traceable to the big bang and to the thermonuclear furnaces within high-mass stars that exploded more than five billion years ago.
Newton had figured out that the force of gravity pulling ripe apples from their orchards also guides tossed objects along their curved trajectories and directs the Moon in its orbit around Earth.
Chemical elements reveal themselves by their unique patterns of light or dark bands that cut across the spectrum. To people’s delight and amazement, the chemical signatures on the Sun were identical to those in the laboratory. No longer the exclusive tool of chemists, the prism showed that as different as the Sun is from Earth in size, mass, temperature, location, and appearance, we both contain the same stuff: hydrogen, carbon, oxygen, nitrogen, calcium, iron, and so forth. But more important than our laundry list of shared ingredients was the recognition that the laws of physics prescribing the formation of these spectral signatures on the Sun were the same laws operating on Earth, ninety-three million miles away.
Further analysis of the Sun’s spectrum revealed the signature of an element that had no known counterpart on Earth. Being of the Sun, the new substance was given a name derived from the Greek word helios (“the Sun”), and was only later discovered in the lab. Thus, helium became the first and only element in the chemist’s Periodic Table to be discovered someplace other than Earth.
Of course, not all things and phenomena in the cosmos have counterparts on Earth. You’ve probably never walked through a cloud of glowing million-degree plasma, and I’d bet you’ve never greeted a black hole on the street. What matters is the universality of the physical laws that describe them.
This universality of physical laws tells us that if we land on another planet with a thriving alien civilization, they will be running on the same laws that we have discovered and tested here on Earth—even if the aliens harbor different social and political beliefs. Furthermore, if you wanted to talk to the aliens, you can bet they don’t speak English or French or even Mandarin. Nor would you know whether shaking their hands—if indeed their outstretched appendage is a hand—would be considered an act of war or of peace. Your best hope is to find a way to communicate using the language of science.
It happens that we cannot see, touch, or taste the source of eighty-five percent of the gravity we measure in the universe. This mysterious dark matter, which remains undetected except for its gravitational pull on matter we see, may be composed of exotic particles that we have yet to discover or identify.
To the scientist, the universality of physical laws makes the cosmos a marvelously simple place. By comparison, human nature—the psychologist’s domain—is infinitely more daunting. In America, local school boards vote on subjects to be taught in the classroom. In some cases, votes are cast according to the whims of cultural, political, or religious tides. Around the world, varying belief systems lead to political differences that are not always resolved peacefully. The power and beauty of physical laws is that they apply everywhere, whether or not you choose to believe in them.
After the big bang, the main agenda of the cosmos was expansion, ever diluting the concentration of energy that filled space. With each passing moment the universe got a little bit bigger, a little bit cooler, and a little bit dimmer.
For household lamps that still use glowing metal filaments, the bulbs all peak in the infrared, which is the single greatest contributor to their inefficiency as a source of visible light. Our senses detect infrared only in the form of warmth on our skin. The LED revolution in advanced lighting technology creates pure visible light without wasting wattage on invisible parts of the spectrum. That’s how you can get crazy-sounding sentences like: “7 Watts LED replaces 60 Watts Incandescent” on the packaging.
Ordinary matter is what we are all made of. It has gravity and interacts with light. Dark matter is a mysterious substance that has gravity but does not interact with light in any known way. Dark energy is a mysterious pressure in the vacuum of space that acts in the opposite direction of gravity, forcing the universe to expand faster than it otherwise would.
Our own spiral-shaped galaxy, the Milky Way, is named for its spilled-milk appearance to the unaided eye across Earth’s nighttime sky.
In any reliably surveyed volume of space, dwarf galaxies outnumber large galaxies by more than ten to one.
In fact, observations of clusters detect just such a glow between the galaxies, suggesting that there may be as many vagabond, homeless stars as there are
Supernovas are stars that have blown themselves to smithereens and, in the process, have temporarily (over several weeks) increased their luminosity a billion-fold, making them visible across the universe.
Like the dinosaurs that came and went, leaving birds as their only modern descendant,
where there is mass there is gravity. And where there is gravity there is curved space, according to Einstein’s general theory of relativity. And where space is curved it can mimic the curvature of an ordinary glass lens and alter the pathways of light that pass through.
We don’t know who’s next in the genius sequence, but we’ve now been waiting nearly a century for somebody to tell us why the bulk of all the gravitational force that we’ve measured in the universe—about eighty-five percent of it—arises from substances that do not otherwise interact with “our” matter or energy. Or maybe the excess gravity doesn’t come from matter and energy at all, but emanates from some other conceptual thing. In any case, we are essentially clueless.
Turns out, if we could boost Earth’s orbital speed to more than the square root of two (1.4142 . . .) times its current value, our planet would achieve “escape velocity,” and leave the solar system entirely.
When we examine the Coma cluster, as Zwicky did during the 1930s, we find that its member galaxies are all moving more rapidly than the escape velocity for the cluster.
Perhaps the “missing mass” needed to bind the Coma cluster’s galaxies does exist, but in some unknown, invisible form. Today, we’ve settled on the moniker “dark matter,” which makes no assertion that anything is missing, yet nonetheless implies that some new kind of matter must exist, waiting to be discovered.
From galaxy to galaxy and from cluster to cluster, the discrepancy between the mass tallied from visible objects and the objects’ mass estimated from total gravity ranges from a factor of a few up to (in some cases) a factor of many hundreds. Across the universe, the discrepancy averages to a factor of six: cosmic dark matter has about six times the total gravity of all the visible matter.
Thus, as best we can figure, the dark matter doesn’t simply consist of matter that happens to be dark. Instead, it’s something else altogether. Dark matter exerts gravity according to the same rules that ordinary matter follows, but it does little else that might allow us to detect it. Of course, we are hamstrung in this analysis by not knowing what the dark matter is in the first place. If all mass has gravity, does all gravity have mass? We don’t know. Maybe there’s nothing wrong with the matter, and it’s the gravity we don’t understand.
What we know is that the matter we have come to love in the universe—the stuff of stars, planets, and life—is only a light frosting on the cosmic cake, modest buoys afloat in a vast cosmic ocean of something that looks like nothing.
At odds in the universe were two competing effects: gravity wants to make stuff coagulate, but the expansion wants to dilute it. If you do the math, you rapidly deduce that the gravity from ordinary matter could not win this battle by itself. It needed the help of dark matter, without which we would be living—actually not living—in a universe with no structures: no clusters, no galaxies, no stars, no planets, no people.
But light turns out to be quite happy traveling through the vacuum of space, devoid of any medium to carry it. Unlike sound waves, which consist of air vibrations, light waves were found to be self-propagating packets of energy requiring no assistance at all.
Science is not just about seeing, it’s about measuring, preferably with something that’s not your own eyes, which are inextricably conjoined with the baggage of your brain. That baggage is more often than not a satchel of preconceived ideas, post-conceived notions, and outright bias.
the strong nuclear force, weak nuclear force, and electromagnetism. These three, plus gravity, complete the fab four forces of the universe, mediating all interactions between and among all known particles.
So the choices are clear. Either dark matter particles must wait for us to discover and to control a new force or class of forces through which their particles interact, or else dark matter particles interact via normal forces, but with staggering weakness.
So, dark matter’s effects are real. We just don’t know what it is. Dark matter seems not to interact through the strong nuclear force, so it cannot make nuclei. It hasn’t been found to interact through the weak nuclear force, something even elusive neutrinos do. It doesn’t seem to interact with the electromagnetic force, so it doesn’t make molecules and concentrate into dense balls of dark matter. Nor does it absorb or emit or reflect or scatter light. As we’ve known from the beginning, dark matter does, indeed, exert gravity, to which ordinary matter responds. But that’s it.
Instead of settling for Sir Isaac Newton’s view of gravity as spooky action-at-a-distance (a conclusion that made Newton himself uncomfortable), GR regards gravity as the response of a mass to the local curvature of space and time caused by some other mass or field of energy. In other words, concentrations of mass cause distortions—dimples, really—in the fabric of space and time. These distortions guide the moving masses along straight-line geodesics,††† though they look to us like the curved trajectories we call orbits.
“Matter tells space how to curve; space tells matter how to move.”
The most accurate measurements to date reveal dark energy as the most prominent thing in town, currently responsible for 68 percent of all the mass-energy in the universe; dark matter comprises 27 percent, with regular matter comprising a mere 5 percent.
Only three of the naturally occurring elements were manufactured in the big bang. The rest were forged in the high-temperature hearts and explosive remains of dying stars, enabling subsequent generations of star systems to incorporate this enrichment, forming planets and, in our case, people.
How else could we believe that sodium is a poisonous, reactive metal that you can cut with a butter knife, while pure chlorine is a smelly, deadly gas, yet when added together they make sodium chloride, a harmless, biologically essential compound better known as table salt? Or how about hydrogen and oxygen? One is an explosive gas, and the other promotes violent combustion, yet the two combined make liquid water, which puts out fires.
With only one proton in its nucleus, hydrogen is the lightest and simplest element, made entirely during the big bang. Out of the ninety-four naturally occurring elements, hydrogen lays claim to more than two-thirds of all the atoms in the human body, and more than ninety percent of all atoms in the cosmos,
Helium is the second simplest and second most abundant element in the universe. Although a distant second to hydrogen in abundance, there’s four times more of it than all other elements in the universe combined.
Lithium is the third simplest element in the universe, with three protons in its nucleus. Like hydrogen and helium, lithium was made in the big bang,
Given the abundance of carbon in the cosmos—forged in the cores of stars, churned up to their surfaces, and released copiously into the galaxy—a better element does not exist on which to base the chemistry and diversity of life. Just edging out carbon in abundance rank, oxygen is common, too, forged and released in the remains of exploded stars. Both oxygen and carbon are major ingredients of life as we know it.
Aluminum occupies nearly ten percent of Earth’s crust yet was unknown to the ancients and unfamiliar to our great-grandparents. The element was not isolated and identified until 1827 and did not enter common household use until the late 1960s, when tin cans and tin foil yielded to aluminum cans and, of course, aluminum foil.
By many measures, iron ranks as the most important element in the universe.
Iridium is rare on Earth’s surface but relatively common in six-mile metallic asteroids, which, upon colliding with Earth, vaporize on impact, scattering their atoms across Earth’s surface. So, whatever might have been your favorite theory for offing the dinosaurs, a killer asteroid the size of Mount Everest from outer space should be at the top of your list.
Personally, I am quite comfortable with chemicals, anywhere in the universe. My favorite stars, as well as my best friends, are all made of them.
In fact, billions of dollars could be saved annually on packaging materials if all shipping boxes and all packages of food in the supermarket were spheres.
For large cosmic objects, energy and gravity conspire to turn objects into spheres. Gravity is the force that serves to collapse matter in all directions, but gravity does not always win—chemical bonds of solid objects are strong. The Himalayas grew against the force of Earth’s gravity because of the resilience of crustal rock. But before you get excited about Earth’s mighty mountains, you should know that the spread in height from the deepest undersea trenches to the tallest mountains is about a dozen miles, yet Earth’s diameter is nearly eight thousand miles. So, contrary to what it looks like to teeny humans crawling on its surface, Earth, as a cosmic object, is remarkably smooth.
The stars of the Milky Way galaxy trace a big, flat circle. With a diameter-to-thickness ratio of one thousand to one, our galaxy is flatter than the flattest flapjacks ever made.
Herschel had discovered the planet Uranus in 1781 and was now exploring the relation between sunlight, color, and heat. He began by placing a prism in the path of a sunbeam. Nothing new there. Sir Isaac Newton had done that back in the 1600s, leading him to name the familiar seven colors of the visible spectrum: red, orange, yellow, green, blue, indigo, and violet. (Yes, the colors do indeed spell Roy G. Biv.) But Herschel was inquisitive enough to wonder what the temperature of each color might be. So he placed thermometers in various regions of the rainbow and showed, as he suspected, that different colors registered different temperatures.†
Herschel knew this, and laid a thermometer outside of the spectrum, adjacent to the red, expecting to read no more than room temperature throughout the experiment. But that’s not what happened. The temperature of his control thermometer rose even higher than in the red. Herschel wrote: [I] conclude, that the full red falls still short of the maximum of heat; which perhaps lies even a little beyond visible refraction. In this case, radiant heat will at least partly, if not chiefly, consist, if I may be permitted the expression, of invisible light; that is to say, of rays coming from the sun, that have such a momentum as to be unfit for vision.†† Holy s#%t! Herschel inadvertently discovered “infra” red light, a brand-new part of the spectrum found just “below” red,
What’s beyond violet? “Ultra” violet, better known today as UV.
Filling out the entire electromagnetic spectrum, in order of low-energy and low-frequency to high-energy and high-frequency, we have: radio waves, microwaves, infrared, ROYGBIV, ultraviolet, X-rays, and gamma rays. Modern civilization has deftly exploited each of these bands for countless household and industrial applications, making them familiar to us all.
These two phenomena are, of course, related: water is the most common ingredient in food, and microwave ovens primarily heat water. Taken together, you get the best indication that water absorbs microwave frequencies.
From a distance, our solar system looks empty. If you enclosed it within a sphere—one large enough to contain the orbit of Neptune, the outermost planet†—then the volume occupied by the Sun, all planets, and their moons would take up a little more than one-trillionth the enclosed space.
At the rate we are discovering meteorites on Earth whose origin is Mars, we conclude that about a thousand tons of Martian rocks rain down on Earth each year. Perhaps the same amount reaches Earth from the Moon. In retrospect, we didn’t have to go to the Moon to retrieve Moon rocks. Plenty come to us, although they were not of our choosing and we didn’t yet know it during the Apollo program.
A simple calculation reveals that most of them will hit Earth within a hundred million years. The ones larger than about a kilometer across will collide with enough energy to destabilize Earth’s ecosystem and put most of Earth’s land species at risk of extinction.
Earth’s Moon is about 1/400th the diameter of the Sun, but it is also 1/400th as far from us, making the Sun and the Moon the same size on the sky—a coincidence not shared by any other planet–moon combination in the solar system, allowing for uniquely photogenic total solar eclipses.
The Sun loses material from its surface at a rate of more than a million tons per second. We call this the “solar wind,” which takes the form of high-energy charged particles. Traveling up to a thousand miles per second, these particles stream through space and are deflected by planetary magnetic fields.
The particles spiral down toward the north and south magnetic poles, forcing collisions with gas molecules and leaving the atmosphere aglow with colorful aurora. The Hubble Space Telescope has spotted aurora near the poles of both Saturn and Jupiter. And on Earth, the aurora borealis and australis (the northern and southern lights) serve as intermittent reminders of how nice it is to have a protective atmosphere.
The planet Jupiter, with its mighty gravitational field, bats out of harm’s way many comets that would otherwise wreak havoc on the inner solar system. Jupiter acts as a gravitational shield for Earth, a burly big brother, allowing long (hundred-million-year) stretches of relative peace and quiet on Earth. Without Jupiter’s protection, complex life would have a hard time becoming interestingly complex, always living at risk of extinction from a devastating impact.
From the window of the International Space Station, which orbits at about 250 miles up, you might find Paris, London, New York, and Los Angeles in the daytime, but only because you learned where they are in geography class. At night, their sprawling cityscapes present an obvious glow. By day, contrary to common wisdom, you probably won’t see the Great Pyramids at Giza, and you certainly won’t see the Great Wall of China.
Consider everything we’ve got that generates radio waves and microwaves: not only traditional radio itself, but also broadcast television, mobile phones, microwave ovens, garage-door openers, car-door unlockers, commercial radar, military radar, and communications satellites. We’re ablaze in long-frequency waves—spectacular evidence that something unusual is going on here, because in their natural state, small rocky planets emit hardly any radio waves at all.
Oxygen—which, after hydrogen and helium, is the third most abundant element in the cosmos—is chemically active and bonds readily with atoms of hydrogen, carbon, nitrogen, silicon, sulfur, iron, and so on. It even bonds with itself. Thus, for oxygen to exist in a steady state, something must be liberating it as fast as it’s being consumed. Here on Earth, the liberation is traceable to life. Photosynthesis, carried out by plants and many bacteria, creates free oxygen in the oceans and in the atmosphere. Free oxygen, in turn, enables the existence of oxygen-metabolizing life, including us and practically every other creature in the animal kingdom.
Looking more closely at Earth’s atmospheric fingerprints, human biomarkers will also include sulfuric, carbonic, and nitric acids, and other components of smog from the burning of fossil fuels. If the curious aliens happen to be socially, culturally, and technologically more advanced than we are, then they will surely interpret these biomarkers as convincing evidence for the absence of intelligent life on Earth.
Our search for life in the universe drives the search for exoplanets, some of which resemble Earth—not in detail, of course, but in overall properties. Latest estimates, extrapolating from the current catalogs, suggests as many as forty billion Earth-like planets in the Milky Way alone.
Part the curtains of society’s racial, ethnic, religious, national, and cultural conflicts, and you find the human ego turning the knobs and pulling the levers.
Now imagine a world in which everyone, but especially people with power and influence, holds an expanded view of our place in the cosmos. With that perspective, our problems would shrink—or never arise at all—and we could celebrate our earthly differences while shunning the behavior of our predecessors who slaughtered one another because of them.
His ego was unjustifiably big to begin with, inflated by delusions of significance and fed by cultural assumptions that human beings are more important than everything else in the universe. In all fairness to the fellow, powerful forces in society leave most of us susceptible. As was I, until the day I learned in biology class that more bacteria live and work in one centimeter of my colon than the number of people who have ever existed in the world. That kind of information makes you think twice about who—or what—is actually in charge. From that day on, I began to think of people not as the masters of space and time but as participants in a great cosmic chain of being, with a direct genetic link across species both living and extinct, extending back nearly four billion years to the earliest single-celled organisms on Earth.
Imagine a life-form whose brainpower is to ours as ours is to a chimpanzee’s. To such a species, our highest mental achievements would be trivial.
Take water. It’s common, and vital. There are more molecules of water in an eight-ounce cup of the stuff than there are cups of water in all the world’s oceans. Every cup that passes through a single person and eventually rejoins the world’s water supply holds enough molecules to mix 1,500 of them into every other cup of water in the world. No way around it: some of the water you just drank passed through the kidneys of Socrates, Genghis Khan, and Joan of Arc.
How about air? Also vital. A single breathful draws in more air molecules than there are breathfuls of air in Earth’s entire atmosphere. That means some of the air you just breathed passed through the lungs of Napoleon, Beethoven, Lincoln, and Billy the Kid.
There are more stars in the universe than grains of sand on any beach, more stars than seconds have passed since Earth formed, more stars than words and sounds ever uttered by all the humans who ever lived.
The chemical elements of the universe are forged in the fires of high-mass stars that end their lives in titanic explosions, enriching their host galaxies with the chemical arsenal of life as we know it. The result? The four most common, chemically active elements in the universe—hydrogen, oxygen, carbon, and nitrogen—are the four most common elements of life on Earth, with carbon serving as the foundation of biochemistry. We do not simply live in this universe. The universe lives within us.
As we’ve already seen, when a large asteroid strikes a planet, the surrounding areas can recoil from the impact energy, catapulting rocks into space. From there, they can travel to—and land on—other planetary surfaces. Second, microorganisms can be hardy. Extremophiles on Earth can survive wide ranges of temperature, pressure, and radiation encountered during space travel. If the rocky ejecta from an impact hails from a planet with life, then microscopic fauna could have stowed away in the rocks’ nooks and crannies. Third, recent evidence suggests that shortly after the formation of our solar system, Mars was wet, and perhaps fertile, even before Earth was. Collectively, these findings tell us it’s conceivable that life began on Mars and later seeded life on Earth, a process known as panspermia. So all Earthlings might—just might—be descendants of Martians.