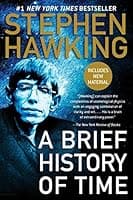
As long ago as 340 B.C. the Greek philosopher Aristotle, in his book On the Heavens, was able to put forward two good arguments for believing that the earth was a round sphere rather than a flat plate. First, he realized that eclipses of the moon were caused by the earth coming between the sun and the moon. The earth’s shadow on the moon was always round, which would be true only if the earth was spherical.
In addition, Newton postulated a law of universal gravitation according to which each body in the universe was attracted toward every other body by a force that was stronger the more massive the bodies and the closer they were to each other. It was this same force that caused objects to fall to the ground.
In an infinite universe, every point can be regarded as the center, because every point has an infinite number of stars on each side of it. The correct approach, it was realized only much later, is to consider the finite situation, in which the stars all fall in on each other, and then to ask how things change if one adds more stars roughly uniformly distributed outside this region.
It was generally accepted that either the universe had existed forever in an unchanging state, or that it had been created at a finite time in the past more or less as we observe it today. In part this may have been due to people’s tendency to believe in eternal truths, as well as the comfort they found in the thought that even though they may grow old and die, the universe is eternal and unchanging.
But in 1929, Edwin Hubble made the landmark observation that wherever you look, distant galaxies are moving rapidly away from us. In other words, the universe is expanding.
Hubble’s observations suggested that there was a time, called the big bang, when the universe was infinitesimally small and infinitely dense.
One could still imagine that God created the universe at the instant of the big bang, or even afterwards in just such a way as to make it look as though there had been a big bang, but it would be meaningless to suppose that it was created before the big bang.
An expanding universe does not preclude a creator, but it does place limits on when he might have carried out his job!
A theory is a good theory if it satisfies two requirements. It must accurately describe a large class of observations on the basis of a model that contains only a few arbitrary elements, and it must make definite predictions about the results of future observations.
Any physical theory is always provisional, in the sense that it is only a hypothesis: you can never prove it. No matter how many times the results of experiments agree with some theory, you can never be sure that the next time the result will not contradict the theory. On the other hand, you can disprove a theory by finding even a single observation that disagrees with the predictions of the theory.
The eventual goal of science is to provide a single theory that describes the whole universe.
Today scientists describe the universe in terms of two basic partial theories—the general theory of relativity and quantum mechanics.
One of the major endeavors in physics today, and the major theme of this book, is the search for a new theory that will incorporate them both—a quantum theory of gravity. We
Darwin’s principle of natural selection. The idea is that in any population of self-reproducing organisms, there will be variations in the genetic material and upbringing that different individuals have.
These individuals will be more likely to survive and reproduce and so their pattern of behavior and thought will come to dominate.
Galileo’s measurements indicated that each body increased its speed at the same rate, no matter what its weight. For example, if you let go of a ball on a slope that drops by one meter for every ten meters you go along, the ball will be traveling down the slope at a speed of about one meter per second after one second, two meters per second after two seconds, and so on, however heavy the ball. Of course a lead weight would fall faster than a feather, but that is only because a feather is slowed down by air resistance. If one drops two bodies that don’t have much air resistance, such as two different lead weights, they fall at the same rate. On the moon, where there is no air to slow things down, the astronaut David R. Scott performed the feather and lead weight experiment and found that indeed they did hit the ground at the same time.
Newton’s second law. This states that the body will accelerate, or change its speed, at a rate that is proportional to the force. (For example, the acceleration is twice as great if the force is twice as great.) The acceleration is also smaller the greater the mass (or quantity of matter) of the body. (The same force acting on a body of twice the mass will produce half the acceleration.) A familiar example is provided by a car: the more powerful
One can now see why all bodies fall at the same rate: a body of twice the weight will have twice the force of gravity pulling it down, but it will also have twice the mass. According to Newton’s second law, these two effects will exactly cancel each other, so the acceleration will be the same in all cases.
Luke's Note
Force of gravity is the same thing as weight
The lack of an absolute standard of rest meant that one could not determine whether two events that took place at different times occurred in the same position in space. For example, suppose our Ping-Pong ball on the train bounces straight up and down, hitting the table twice on the same spot one second apart. To someone on the track, the two bounces would seem to take place about forty meters apart, because the train would have traveled that far down the track between the bounces. The nonexistence of absolute rest therefore meant that one could not give an event an absolute position in space, as Aristotle had believed. The positions of events and the distances between them would be different for a person on the train and one on the track, and there would be no reason to prefer one person’s position to the other’s.
If the wavelength of these waves (the distance between one wave crest and the next) is a meter or more, they are what we now call radio waves. Shorter wavelengths are known as microwaves (a few centimeters) or infrared (more than a ten-thousandth of a centimeter). Visible light has a wavelength of between only forty and eighty millionths of a centimeter. Even shorter wavelengths are known as ultraviolet, X rays, and gamma rays.
The fundamental postulate of the theory of relativity, as it was called, was that the laws of science should be the same for all freely moving observers, no matter what their speed.
all observers should measure the same speed of light, no matter how fast they are moving. This simple idea has some remarkable consequences. Perhaps the best known are the equivalence of mass and energy, summed up in Einstein’s famous equation E=mc2 (where E is energy, m is mass, and c is the speed of light), and the law that nothing may travel faster than the speed of light.
Because of the equivalence of energy and mass, the energy which an object has due to its motion will add to its mass. In other words, it will make it harder to increase its speed.
As an object approaches the speed of light, its mass rises ever more quickly, so it takes more and more energy to speed it up further. It can in fact never reach the speed of light, because by then its mass would have become infinite, and by the equivalence of mass and energy, it would have taken an infinite amount of energy to get it there. For this reason, any normal object is forever confined by relativity to move at speeds slower than the speed of light. Only light, or other waves that have no intrinsic mass, can move at the speed of light.